When we look out into deep space, beyond the confines of the Milky Way, we find that the Universe isn’t quite so empty. Galaxies — small and large, near and far, in rich clusters and in near-total isolation — fill the abyss of space, with the Milky Way being just one of approximately two trillion such galaxies within the observable Universe. Galaxies are collections of normal matter, including plasmas, gas, dust, planets, and most prominently, stars. It’s through the examination of that starlight that we’ve learned the most about the physical properties of galaxies, and been able to reconstruct how they came to be.
In general, there are four classes of galaxies that we see. Spirals, like the Milky Way, are the most common type of large galaxy in the Universe. Ellipticals, like M87, are the largest and most common type of galaxy in the rich, central regions of galaxy clusters. Irregular galaxies are a third ubiquitous type, usually distorted from a prior spiral or elliptical shape by gravitational interactions. But there’s a very rare type that’s striking and beautiful: ring galaxies. They make up only 1-in-10,000 of all the galaxies out there, with the first one, Hoag’s object, only discovered in 1950. After more than 70 years, we’ve finally figured out how the Universe makes them.
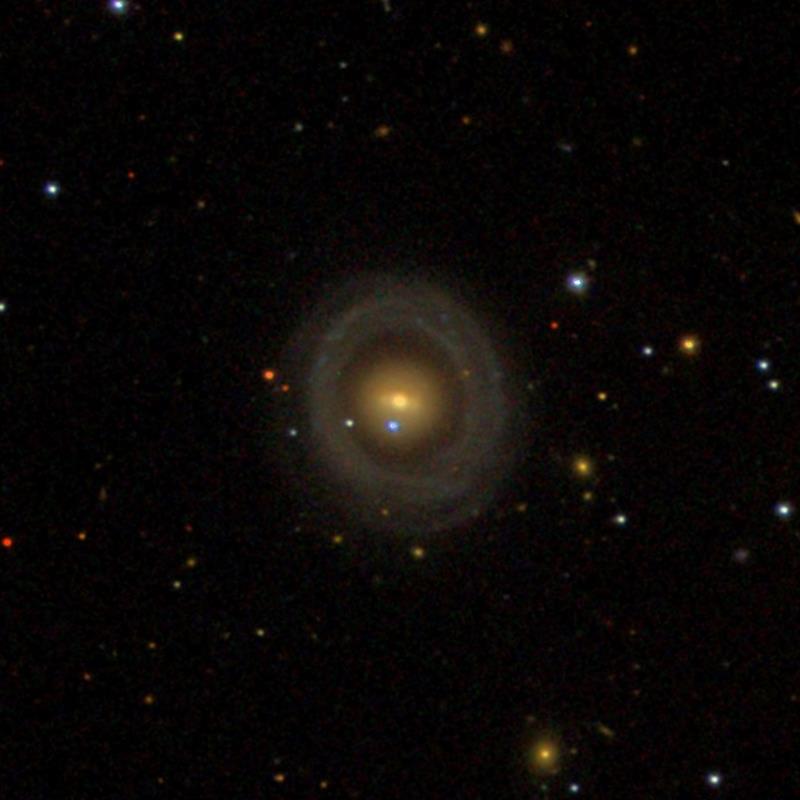
Visually, when you look at a ring galaxy, there’s a set of features that sticks out as unusual among galaxies.
- There’s a central core to the galaxy, relatively compact, that’s low in gas and consists primarily of older stars. There has been very little recent star-formation in that central region.
- Surrounding that galaxy, there’s a gap: a region of very low density, with almost no stars, no light, and very little gas or neutral matter.
- And then, beyond that, there’s another luminous population of stars. This population exists in a bright, luminous ring that surrounds the central core, but is much bluer in color than the core itself. This indicates that the stars within the ring formed much more recently, and are dominated by hot, short-lived, blue colored stars.
In addition, when you look at where ring galaxies are located, they’re overwhelmingly located in what astronomers call “the field,” as opposed to the central locations of rich galaxy groups and clusters. Although this set of features might seem bizarre and unrelated, they’re all cosmic clues to the origins of these features.
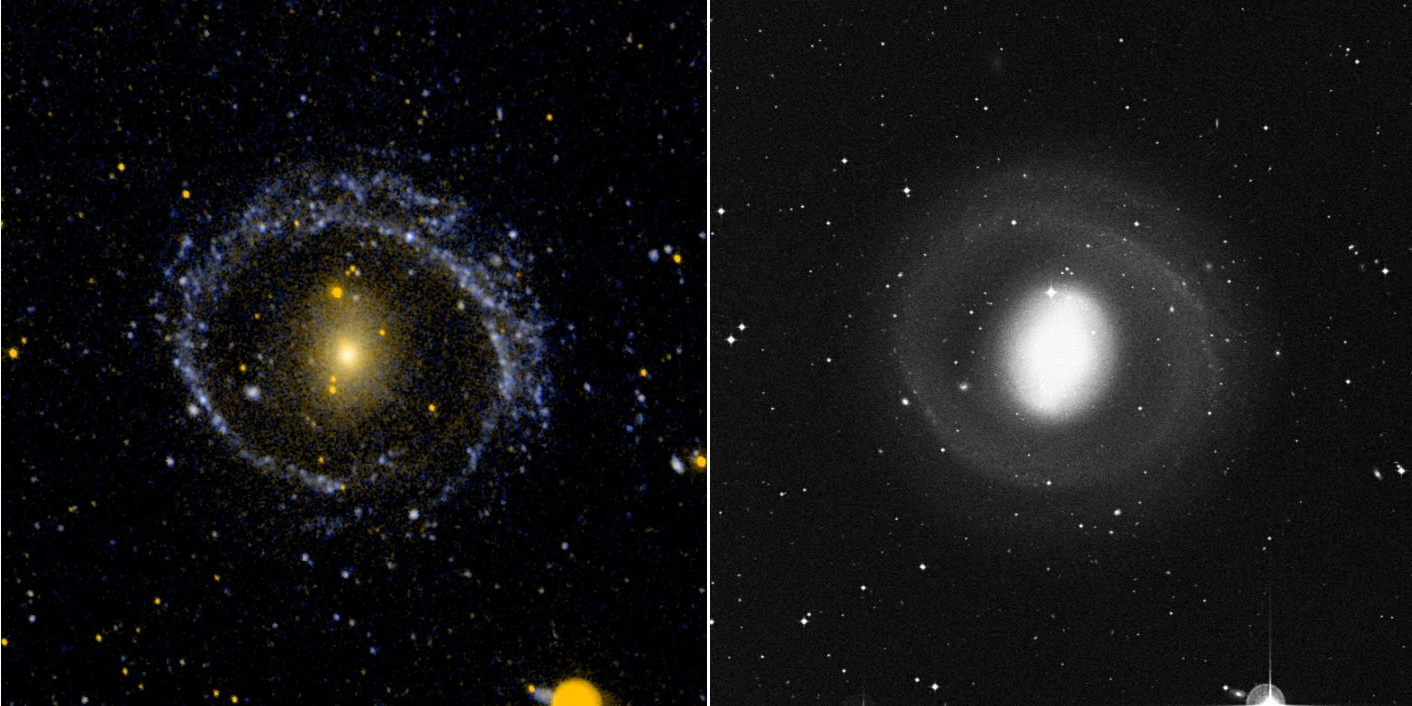
There have been a number of possible explanations put forward for these ring galaxies that we’re certain are wrong, as they cannot account for the observed features when we examine them in detail.
- They aren’t planetary nebulae, which sometimes possess rings around them, as they’re definitively composed of stars, not of gas and other ejecta originating from a single, dying star.
- They aren’t made from a young galaxy getting stretched and ripped apart into a ring that comes to surround a separate, older, more massive galaxy that sits at the center. The ages of the stars in the outer rings and the shapes of the rings themselves show this cannot be the case, as the timescales and angular momentum constraints are in conflict with this possibility.
- And they aren’t examples of gravitational lensing, where a large, massive object stretches, distorts, and magnifies the background light from luminous objects along the same line-of-sight. Gravitational lenses do exist, and can create ring-like shapes under properly aligned conditions, but these ring galaxies all have the “ring” population and the “central” population occurring at the same redshift, ruling out the possibility of a gravitational lens.
Whatever we’re looking at, we can be confident that these are all examples of a single galaxy with two distinct populations of stars: and old one in the central region, and a young one in the ring region.
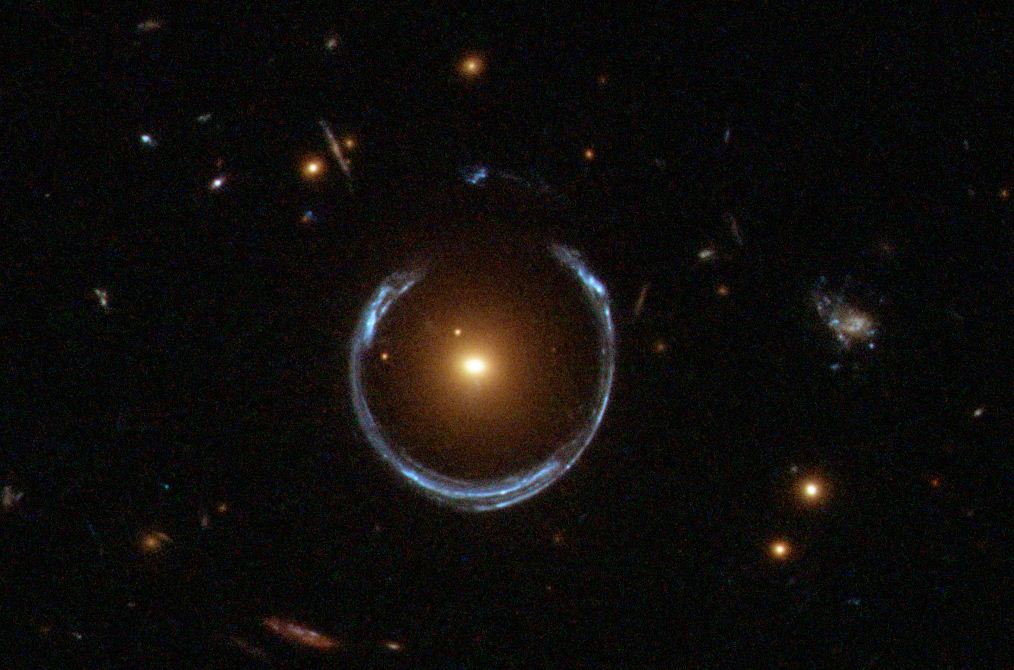
Fortunately, we have a number of examples of these ring galaxies at present, rather than just a single example. By examining their various features, we can put together some of the puzzle pieces, and attempt to assemble a coherent understanding of how these objects form, and explain why they appear with the features and properties that we see.
In April of every year, NASA and the Space Telescope Science Institute always release an anniversary image from Hubble, commemorating its 1990 launch on April 24. Although 2022’s image, celebrating Hubble’s 32nd birthday, is “merely” a tightly-knit galaxy group, the image released for Hubble’s 14th anniversary, back in 2004, provides a series of major clues.
Shown below, galaxy AM 0644-741 reveals a ring that isn’t in a perfectly circular shape, but rather makes a sort-of elongated ellipsoid. In theory, this could either be because there’s a projection effect, and we’re seeing a circular feature as though it’s inclined to us, or because whatever occurred to form the outer ring happened in an asymmetric fashion. As it turns out, both explanations have merit for this one object, but other features are worth pointing out as well.
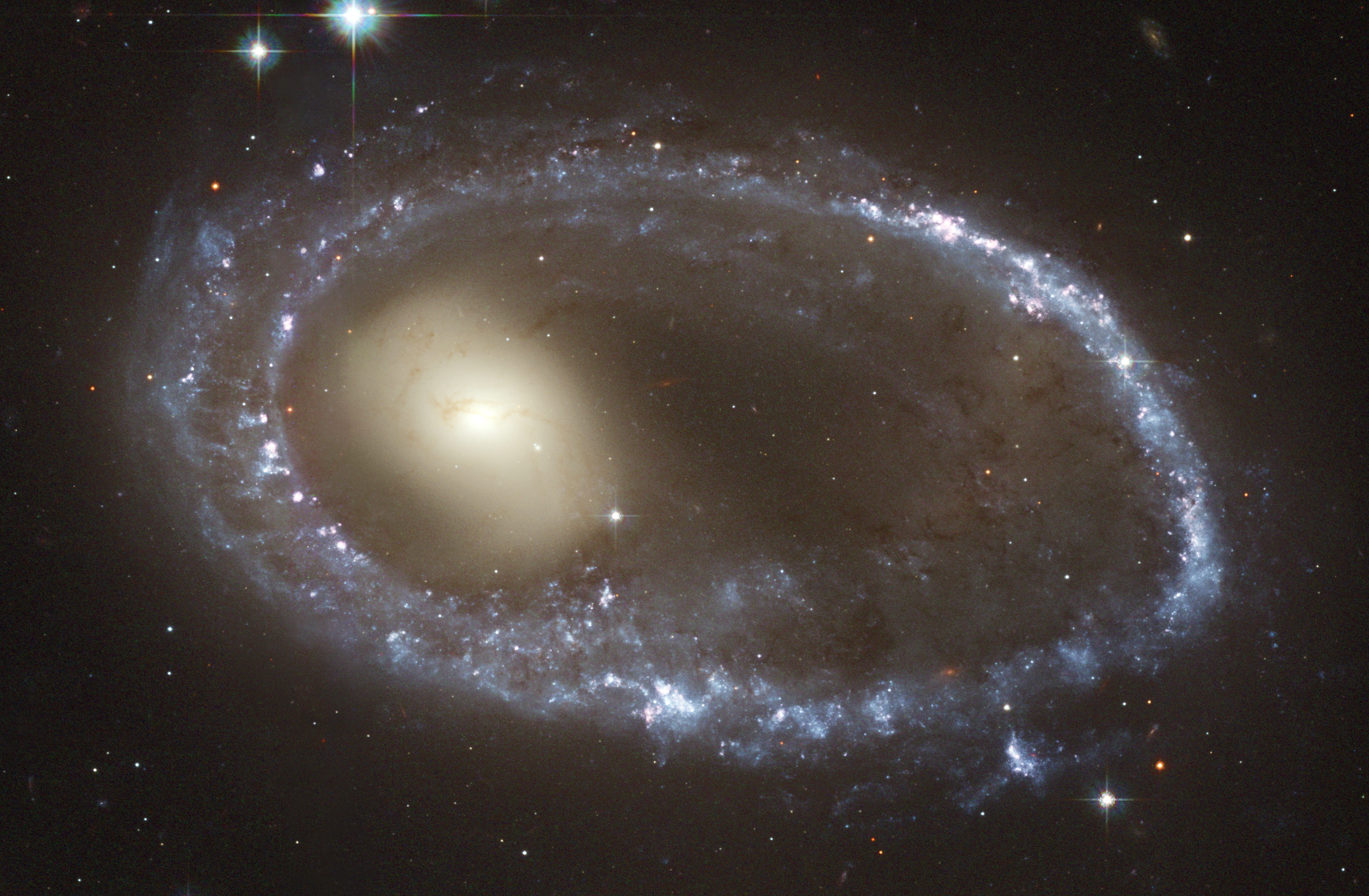
First off, at a distance of only 300 million light-years, it’s relatively easy to resolve a number of important properties. The long axis of the blue-colored ring feature is around 130,000 light-years, making it comparable in size to the Milky Way, while the central, white/yellow-colored component is much smaller at only ~50,000 light-years.
Second, there are dusty features seen silhouetted against the large ringed feature, which shows that not only is there “fuel” remaining to supply gas for continued star-formation, but indicate that there are unequal regions of density inside. Many of the darkest patches are regions which should form new stars moving ahead millions of years into the future.
Third, there are pinkish regions littering the blue ring, which indicate the presence of ionized hydrogen: a typical feature of new star-forming regions where stars are actively being born right now.
And finally, if we look at a wider-field view than the one captured by Hubble, we can even find the culprit: an intruder galaxy that apparently “punched through” what’s now a ring galaxy. In other words, this ring feature didn’t arise out of nowhere, but was caused by an interloper that led to its formation quite recently.
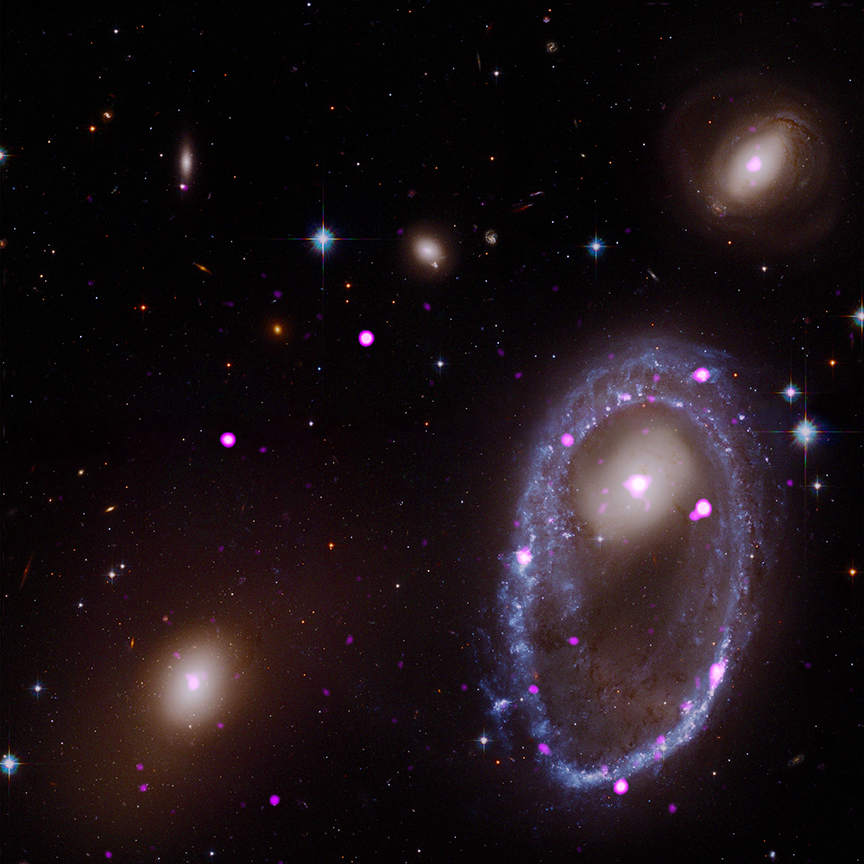
How would this occur? Inside pretty much every spiral galaxy, even in modern times, there are copious reservoirs of gas. Gas gets stripped and depleted, primarily inside rich galaxy clusters, leading to that we call “red and dead” galaxies.
Whenever new stars are formed, those new stars span the full gamut of colors and masses: from hot, blue, and heavy to cool, red, and light. However, the hottest, bluest, most massive stars burn through their fuel the fastest, so they’re the first to die. As a stellar population ages, it goes from blue to white to yellow to orange to red, and the longer it’s been since its last star-formation episode, the redder it is. If there’s no gas left to form new stars, it’s not just red, it’s also “dead,” at least in an astronomical sense.
This is why, we think, we primarily find ring galaxies in the field, rather than in clusters. We need a gas-rich spiral galaxy to start with, and then when an interloping galaxy passes through its center, that collision creates outward-moving ripples in the gas, which trigger star formation and create the notorious ring-like shape.
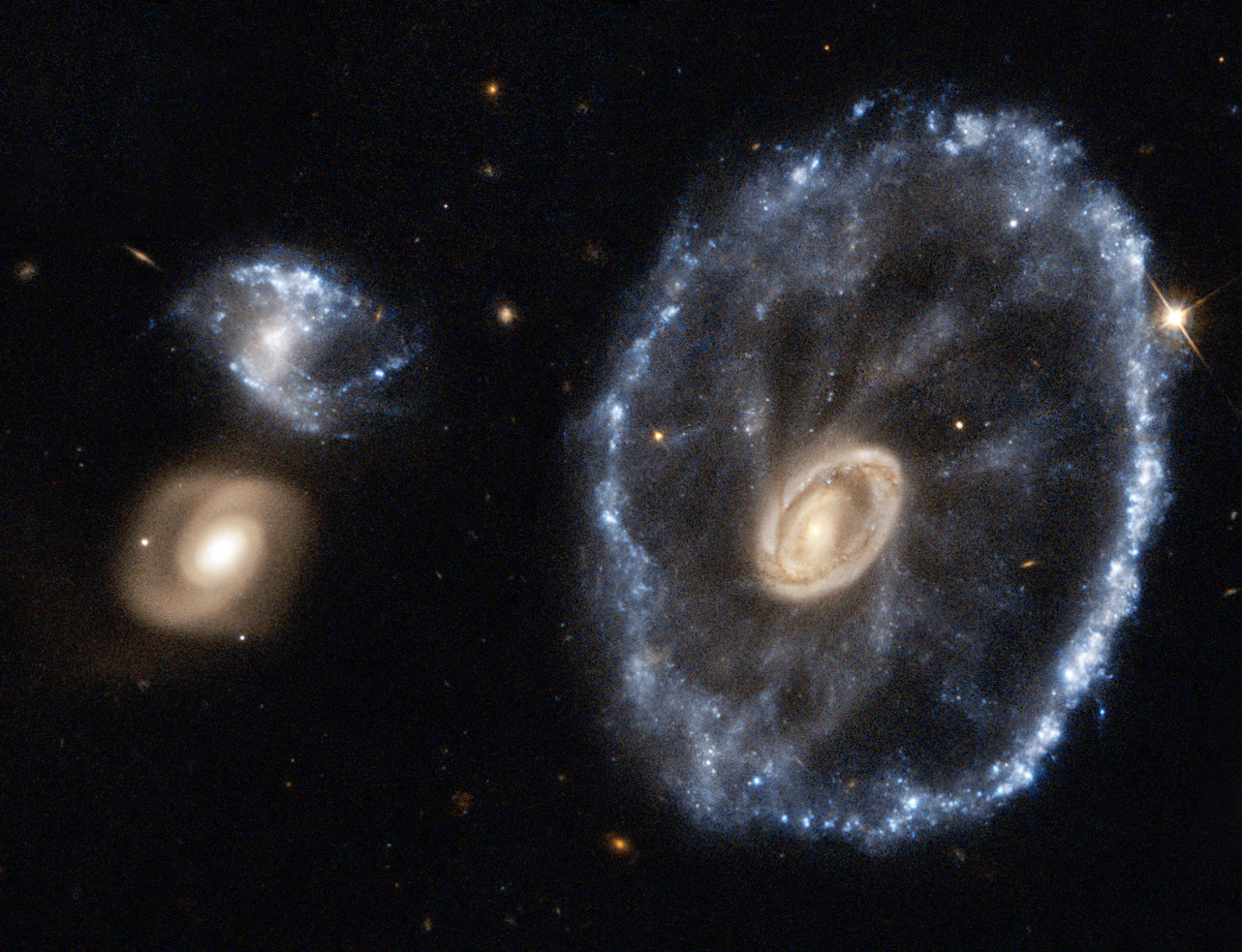
Another example of a ring galaxy, and one that’s clearly in a less-fully-evolved state, is the Cartwheel galaxy, shown above. On the right, you can not only see the dense, older core of a pre-existing gas-rich spiral galaxy surrounded by a bright blue ring of hot, young stars, but also a series of filaments between the core and the ring. Those filaments themselves are dotted with blue and white stars, although of a much lower brightness than either the main core or the ring itself.
Could this have formed in the same fashion: from an interloping galaxy that punched through the center of what’s now a ring galaxy, causing gas to ripple outwards, compress and rarify in turn, and form new stars?
Not only is that the best explanation, but there’s a “smoking gun” just to the left of the Cartwheel galaxy: a smaller, irregular galaxy that itself is rich in young, blue, glittering stars. In other words, in this instance, not only was the Cartwheel galaxy a gas-rich spiral, but so, quite likely, was the interloper, which became irregular owing to the recent interaction.
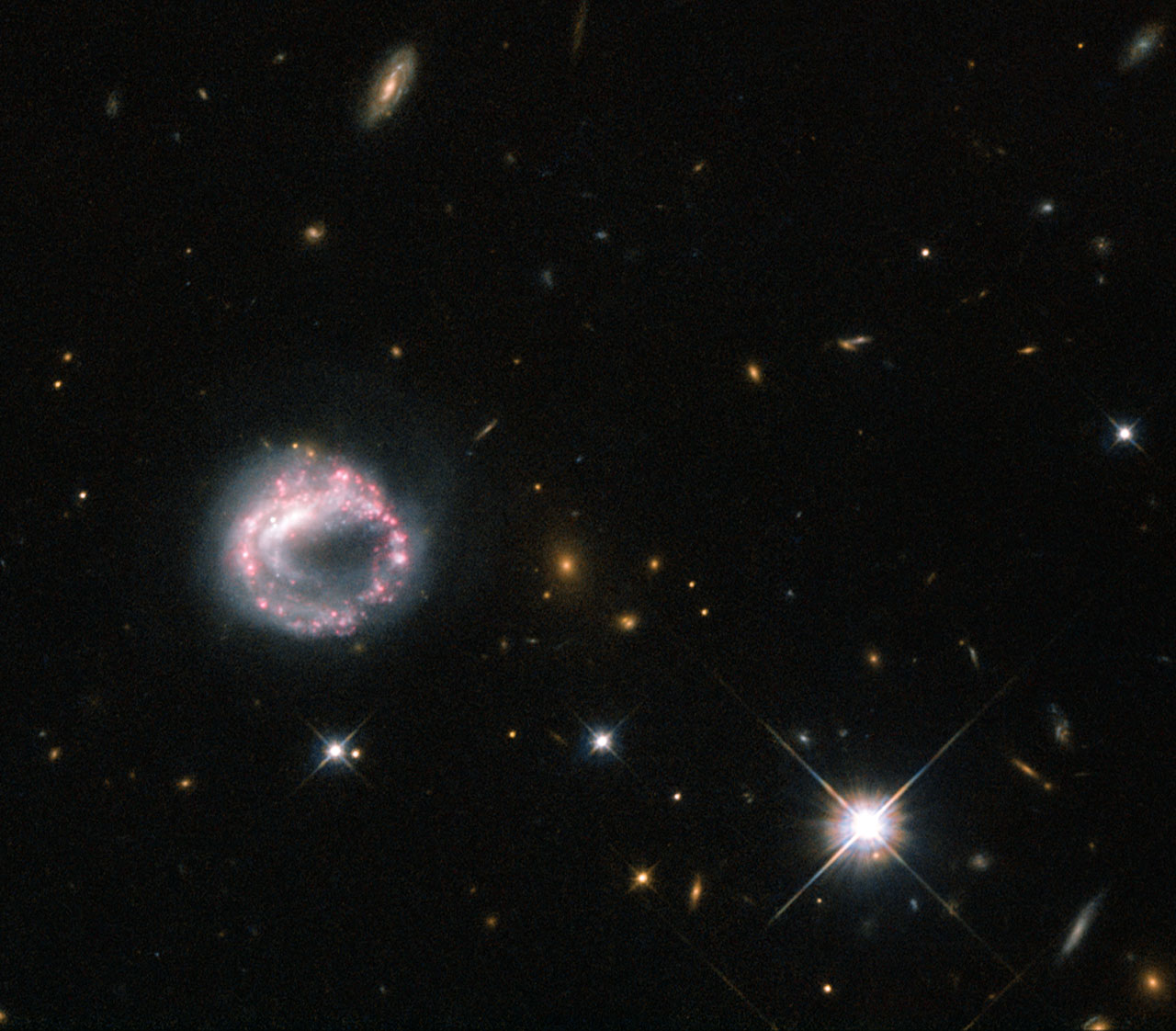
Some ring galaxies, like Zwicky II 28, shown above, are atypical in some fashion. In some cases, the interloper galaxy is nowhere to be found, which is part of why the original ring galaxy — Hoag’s object — remains so mysterious. Others, like this one, appear to lack a central, old core. However, we have to remember, when we look at any one particular object, we’re constrained by our particular perspective. In the case of Zwicky II 28, the asymmetry of the ring is key; the “brighter” part at the top left appears to house the central core, while the “darker” part at the bottom right is antipodal to the core.
In other words, orientation matters!
But it isn’t only orientation; it’s also possible for the entire galaxy, itself, to get stretched into a ring owing to a collision. Generally, this occurs when you have a collision between two very massive galaxies, but one of them initially was relatively low in the number of stars it possessed. It’s then that a collision can lead to both a ring and also to the gravitational disruption of the galaxy itself, allowing both the precursor galaxy and the ring itself to occupy the same region in space. That, rather than a simple displaced core, is likely the cause of at least some coreless ring galaxies, including the one found in Arp 147, below.
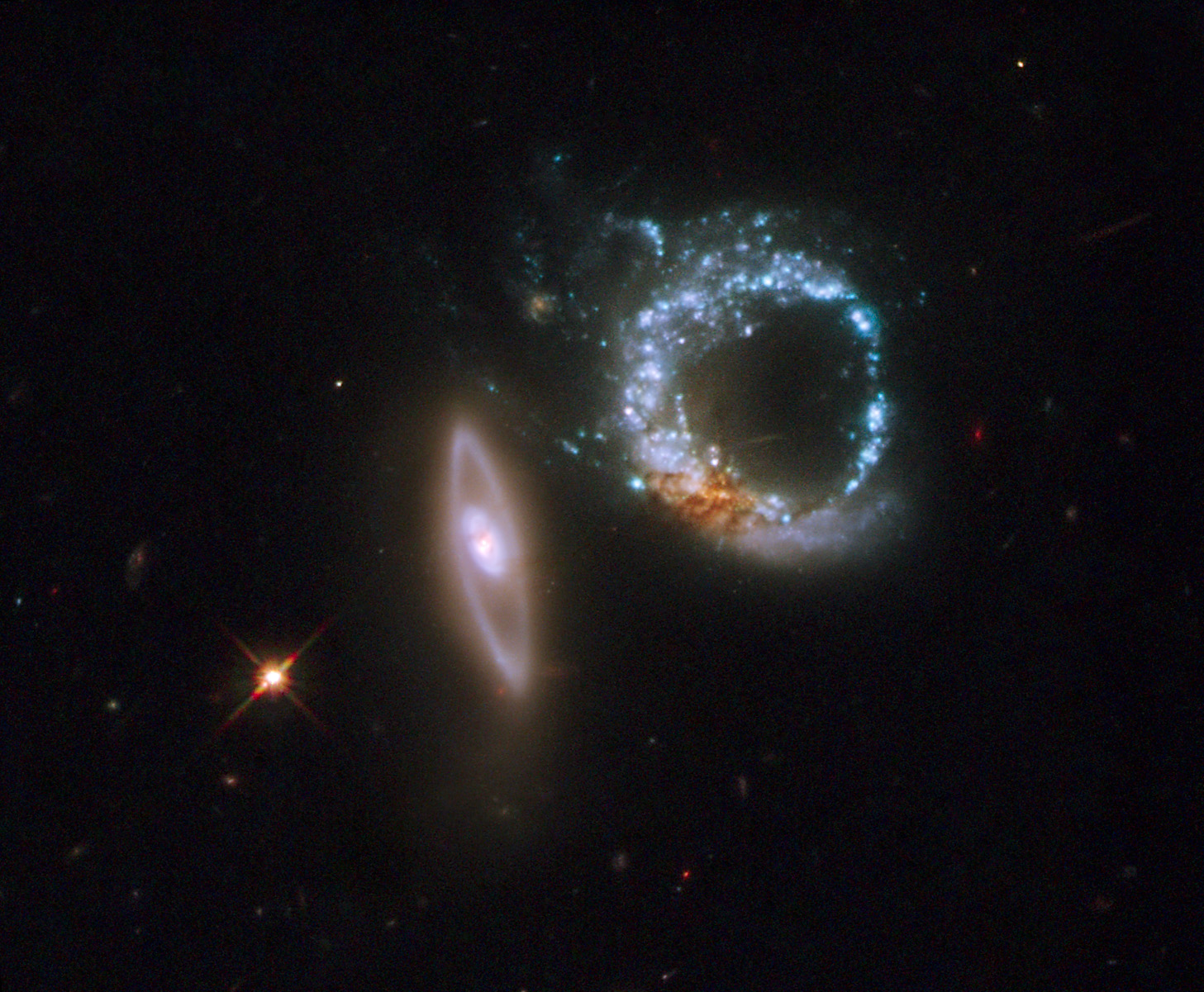
All of this is a very nice story, of course, but are we sure that it’s correct?
There’s one way to put it to the test. In theory, if our picture is right, then we should find:
- pairs of galaxies that speed towards each other and are about to interact,
- a few such pairs where one of them comes in at just the right angle to “punch through” the precise center of the other,
- leading to new stars forming in a ring outside the main galaxy,
- including the possible displacement of part or even all of the original core,
- followed by further evolution into a variety of ring-like shapes, particularly if our sample is large enough.
Simulations can reproduce this, but if we want to confirm it, we need to find examples of all the different stages of this process out there in the Universe. When we observe the Universe, the timescale of human civilization is too short to watch this process unfold; we can only acquire snapshots. We see plenty of examples of interacting pairs of galaxies, particularly in the field (rather than in clusters), with properties that could lead to a ring. And we see many examples of rings themselves, arising from a post-collisional state.
But there are also objects that show the exact critical moment we’d hope to identify, such as Mayall’s object. Originally thought to be a “question mark” when it was first identified in 1940, it is now known to be the collision of two galaxies in the process of creating a ring galaxy.
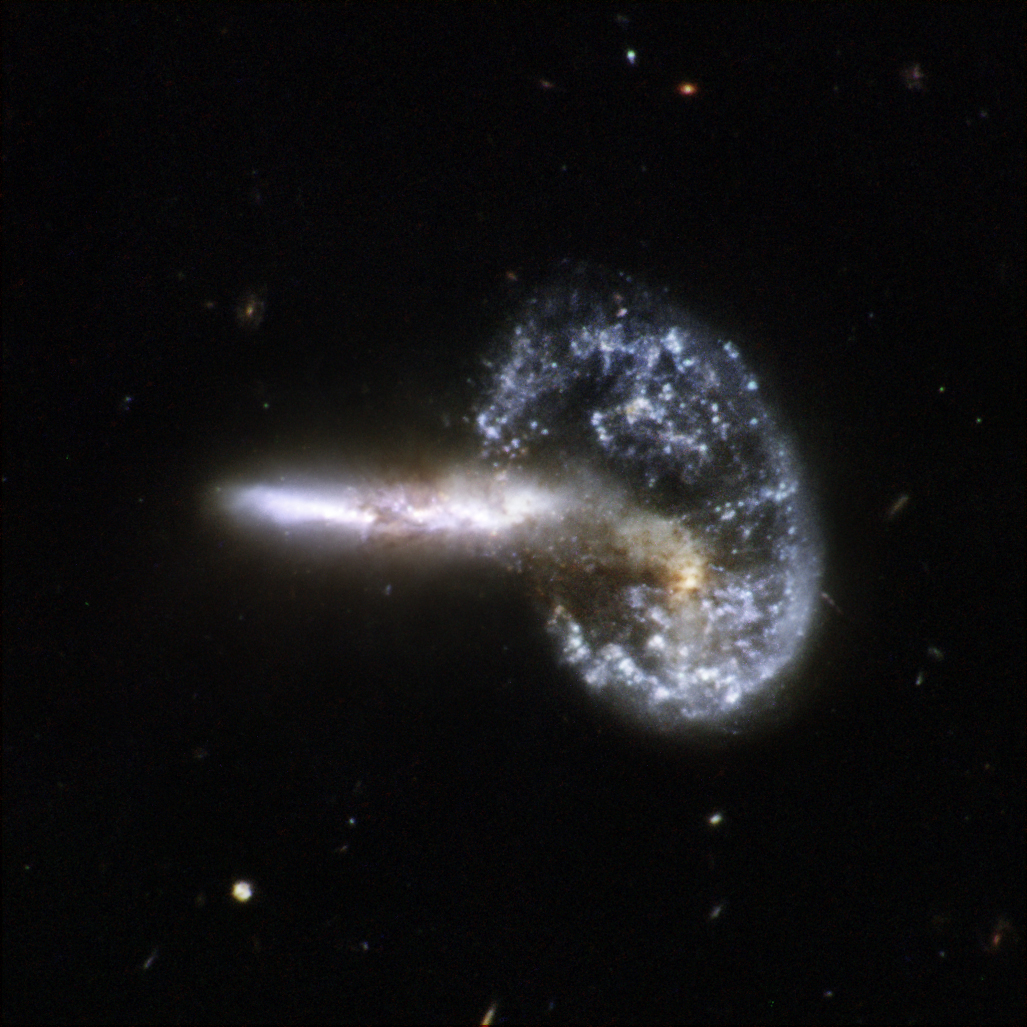
Still, despite the fact that we now know how ring galaxies form in general, Hoag’s object — the original ring — is still an outlier that stubbornly refuses to be explained by any one simple scenario. The ring and the core have almost identical velocities, indicating that if there was an interloper that formed the ring, it was a very quite process. There’s no evidence anywhere in its vicinity for a candidate interloper galaxy, which is surprising, nor are there any galaxy fragments. You can’t save the scenario by pushing the collision farther back into the past, as the outer ring of stars is too young. And the inner core, rather than being a spiral, is instead a gas-poor elliptical.
Still, it’s a remarkable achievement to, on the whole, be able to explain the process by which the rarest class of all major galaxy types, the ring galaxies, form. If you have a gas-rich spiral galaxy and another galaxy can come along and punch right through your center, your internal gases will ripple out towards the edges, smashing into the pre-existing gas along the way, triggering new waves of star-formation on the outskirts, all while depleting the matter in the galactic core. With better data across more wavelengths, the remaining mysteries may yet be solved. Still, it’s always important to appreciate just how far we’ve come in our understanding of not only what’s out there in the Universe, but how what’s out there came to be.
This article was reprinted with permission of Big Think, where it was originally published.